RESOURCE FOR
TIME-RESOLVED LASER SPECTROSCOPY
FEMTORESEARCH.ORG
Absorption Spectroscopies
At its heart, excited state absorption spectroscopy is a technique in which two beams of light to interact with the sample under study. The first is a string of short pulses which populate excited states in the sample, and the second is used to probe transitions from these newly populated excited states. Whether the probe beam is continuous or pulsed, by monitoring the intensity of the probe beam at different times after excitation, the dynamics of the excited states can be directly measured.
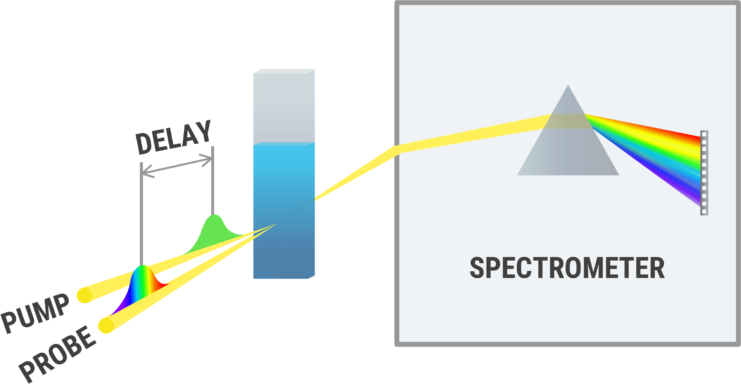
The interaction of the probe beam with short-lived excited states is most often described by the change in absorption as a function of time and probe wavelength:
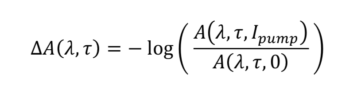
Where 𝐴 = 𝐼0(𝜆). (1 − 10−𝛼𝐿) is the absorbed intensity of each component of the probe through a sample of length L. When both the pump and probe beams are pulsed, this is easily measured by blocking alternate pump pulses and then recording the intensity of the probe with and without prior excitation.
The advantage of monitoring the change in absorption, rather than directly analysing the probe spectrum after the sample, is that the spectral features of the probe, ground state absorption of the sample, and spectral response of the measurement system are removed. This allows for much simpler data analysis, and makes possible direct comparison of pump-probe measurements of different samples taken on different systems.
The pump-probe technique can be divided into four main variants that are routinely performed, with lines of division along the accessible spectral (vis-NIR or IR) and temporal (femtosecond to nanosecond, or longer than nanosecond) ranges of each.
While the general principle of pumping and then probing always applies, the equipment and complexity of each measurement varies considerably. More details on each type of experiment are given on the next pages, with details on suitable pump and probe pulse sources, methods for changing the delay, and suitable detectors.
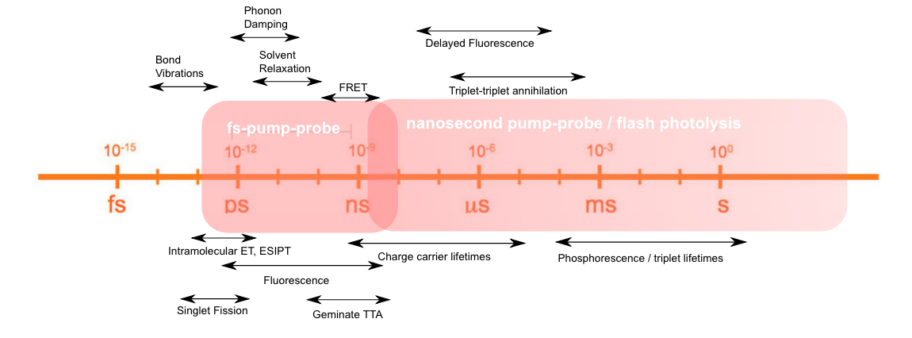
Femtosecond Pump-Probe
The general layout of a femtosecond pump-probe system is described below. Since for sub-picosecond resolution there are no detectors (except perhaps some streak cameras operating in the visible) that can measure single snapshots of the system. This requires pulsed pump and probe light from mode-locked femtosecond oscillators to be used.
Pulses emitted from a laser are split into two beams, and the distance over which one of the beams must travel is varied. Every micrometre added to the path length corresponds to ~3.34 femtosecond delay in arrival time of the pulse, so by mounting a pair of mirrors or a retroreflector to a computer-controlled translation stage, the delay between pulses can be scanned over several nanoseconds with resolution of just a few femtoseconds. The total measurement window is determined by the length of the stage being used, and with a single pass over a 60 cm stage opening up a 4 ns window. This can of course be extended to 8 or even 16 ns by routing the beam over the stage multiple times.
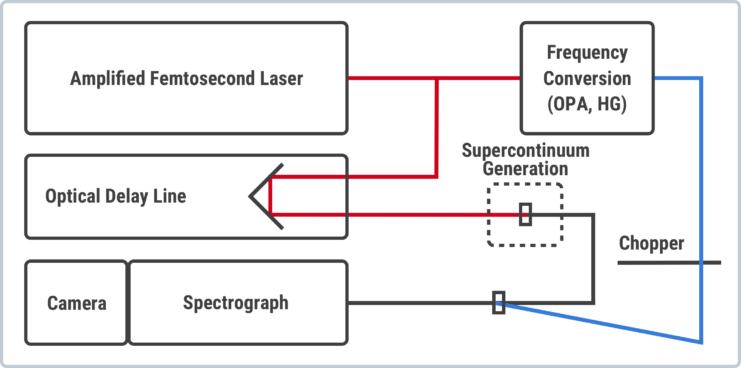
Separating the two beams also allows for the pump and probe wavelengths to be modified before they reach the sample. For single wavelength measurements, the fundamental laser wavelength can be used as both pump and probe, but more often the pump is converted to a higher harmonic by nonlinear crystals placed in the beam. Further tunability of either the pump, probe, or both, can be achieved with either an optical parametric oscillator or amplifier. These are devices in which multiple passes through non-linear crystals allow the output beam to be tuned over a wide range of wavelengths. Modern parametric amplifiers can provide complete tunability from below 300 nm to 20 μm, with good beam quality and similar pulse widths to the input.
Multiple probe wavelengths can also be measured simultaneously by generating a supercontinuum pulse, providing the output from the laser is high enough in energy. When an 800 nm/100 fs pulse of around 1 uJ is focussed into a high-bandgap material, self-phase modulation results in strong broadening of the input pulse. The most commonly used materials for this are sapphire or undoped YAG, and result in a spectrum stretching from 450 nm to 1200 nm. If measurement further into the UV is required then CaF2 can also be used, but the crystal must be moved continuously to avoid permanent damage.
The generated spectrum is dependent on several factors including: the crystal material, crystal length, pump wavelength, pump energy, and focussing conditions. As a general rule, higher pump energies and smaller numerical apertures (NA) will increase the high- energy components generated, while longer crystals and larger NA will generate continua with increased contribution from longer wavelengths.
For excitation and detection with single wavelengths, the pump frequency is usually set to a harmonic of the fundamental. The probe light is then set either to the fundamental, or another value accessible with an optical parametric oscillator or amplifier. Additional flexibility in pump-probe conditions can of course be achieved through the use of a second OPO/OPA, as then both pump and probe wavelengths can be independently tuned.
Since excited state absorption measurements are usually represented as the ratio of absorbances with and without the effect of the pump, this requires a method of blocking every other pump pulse incident on the sample. Mechanical choppers are electric motors with a metal disc in which slots are cut, and they can be synchronised to half the frequency of the main laser. Electro-optic or acousto-optic modulators can also be used to block the pump pulses and may work more efficiently for high repetition rate lasers than mechanical choppers. While these devices are not 100% efficient at blocking pulses like mechanical choppers are, they have no moving parts and so do not suffer from fluctuations in motor speed that can result in mismatch between the laser and chopper frequencies at high repetition rates.
At the sample, both beams are focussed to a spot of 100-200 um in order to increase the total power density. The pump spot must always be kept larger than the probe for accurate measurements and increased signal. Since the pump energies are quite high in pump-probe measurements, photodegradation of the material is a common issue. Solution phase samples are usually cycled through a flow cell so that new material is presented for each pump pulse in order to minimise this effect. Mechanical agitation of the material inside a cuvette is also possible and achieves the same result.
Other samples may require measurements to be performed at cryogenic temperatures, under vacuum, or in the presence of an inert gas. Using specially designed cryostats or holders is therefore required and can impose restrictions on the design of an experiment and achievable time resolution.
After interaction with the sample, the modulated intensity of the probe pulse must be measured. A grating monochromator and detector work to reject scattered pump light and fluorescence from the sample while measuring the probe light. Shot-to-shot fluctuations in intensity of the probe pulse add to the noise of the measurement, however. A second monochromator and detector can be used to minimise this noise by measuring the intensity of part of the probe beam, which has either been diverted around the sample entirely, or passes through part of the sample separated from the pump beam.
With high bandwidth detectors it is possible to measure the intensity of each shot even at high repetition rates (> 100 kHz), but using a lower-bandwidth detector and a lock- in amplifier is also a possibility.
When the full-spectrum is to be recorded each shot the final slit in the monochromator is replaced with a linear or 2-dimensional CCD or CMOS array. 2D arrays allow for higher intensities per pulse to be measured, as vertical summation over multiple pixels is possible, unlike on a linear array. Additionally, if the probe and reference beams are vertically displaced, a single detector can be used to measure both. This comes, however, at a cost of much reduced read speeds for full 2D frames compared with linear arrays. Signals of ΔA < 0.1 mOD can be successfully recorded with full-spectrum probe- reference measurements, and signals of ΔA ~ 0.1-1 uOD are accessible with single colour probe measurements.
Nanosecond Pump-Probe
Pump-probe techniques that measure delays of longer than a few nanoseconds are often referred to as flash-photolysis techniques for historical reasons. When first developed in the 1940s, it involved using a high energy pulses from flashlamps to break apart various gases and measure the absorption spectra of the transient species produced.
The appearance of a modern flash-photolysis experiment is much simpler than that of an ultrafast pump-probe experiment. There is no chopper or optical delay line, however the pump and probe beams must come from two separate light sources. While the pump must always be pulsed, and is the main factor in determining the time resolution, the probe can be either pulsed or continuous, monochromatic or broadband.
In the case of pulsed pump and probe light, the time resolution of the system is limited by the duration of pulse, and also by jitter in the arrival time of each pulse at the sample. Usually the pump laser is set to run at a particular frequency, and an external digital-delay generator is triggered by an electrical output from the laser controller. It then re-emits a second pulse at a specific time after the trigger pulse, which prompts formation and emission of a pulse from the probe source.
These pulses then interact with the sample, and are measured with a monochromator and detector. As with the ultrafast pump-probe technique, either single photodiodes, linear arrays, or 2D CCD/CMOS arrays can be used depending on whether the probe is single wavelength or broadband. The speed of the detector is not important, as only the total intensity of each probe pulse needs to be recorded.
When the probe light is CW, a single high bandwidth detector attached to an oscilloscope will measure an entire transient at a fixed wavelength. The time resolution for this kind of system is most often limited by the detector bandwidth, sensitivity, and the intensity of the probe light. For measurements with ~1 ns time resolution, each individual data point involves measurement of only 1/1,000,000,000th of the total intensity incident on the sample. Unfortunately, the probe powers usually required for high bandwidth detectors to resolve transients on a nanosecond timescale will quite often cause damage the sample! Additionally, the fluorescence of many materials is much more intense than the probe light at short times after excitation and can obscure induced absorption. In practice, CW probe light only really becomes useful for experiments on longer (microsecond to millisecond) timescales.
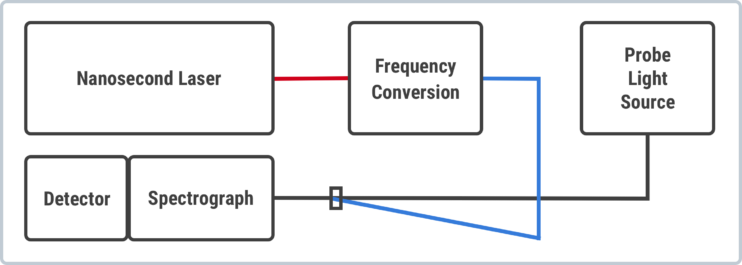
Copyright © 2019 Femtoresearch | All Rights Reserved