RESOURCE FOR
TIME-RESOLVED LASER SPECTROSCOPY
FEMTORESEARCH.ORG
Emission Spectroscopies
Whereas absorption spectroscopy probes transitions from lower to higher energy states, emission spectroscopy looks at radiative transitions back from higher to lower energy states. The techniques are therefore complementary and can give different information about excited and transient states in a system under investigation.
In many ways, emission spectroscopy is a much simpler measurement to both perform and analyse than absorption spectroscopy. A single pulse is used to excite the sample, and the intensity of emission is then directly measured at various times after the initial excitation. Features in the spectrum or the rate at which emission decays can be used to understand the physical processes occurring in the sample, including solvation effects, energy transfer, proton or charge transfer processes, and intramolecular relaxation processes.
Only emissive states are directly measured here, so there are no complications from non-emissive states that may be formed. This can be a complicating factor in time- resolved absorption spectroscopy, and so using both techniques together can be helpful for fully understanding the physics of a sample.
Additionally, states which are formally optically inaccessible (e.g. triplets) can be observed by measuring the long-lived phosphorescence.
As with time-resolved absorption measurements, there are many techniques used to measure emission. Each covers different temporal and spectral ranges, with varying degrees of sensitivity.
The simplest detection method is a fast photodiode or PMT coupled to an oscilloscope which allows direct measurement of the intensity at a single wavelength as a function of time. The minimum temporal resolution is limited by the bandwidth of the detector however, and measurement of the full spectrum requires stitching together multiple decay transients.
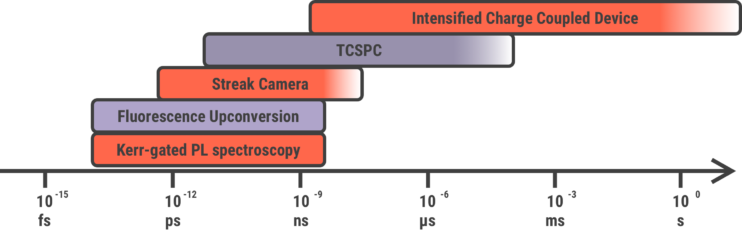
More sophisticated techniques afford higher temporal resolution, with some permitting the entire spectrum to be measured at once. In order to measure with a sub-picosecond resolution, optical gating is required, while for lower resolution, electronic methods work better.
PL Upconversion Spectroscopy
Photoluminescence upconversion spectroscopy is a technique that was developed in the late 80s and still gives the highest resolution for measuring emission spectra. By combining the fluorescence emission and a high energy (gating) pulse from a laser in a crystal with non-linear optical properties, photons with energy equal to the sum of the PL and gate pulses. As this process only occurs when both pulses are overlapped temporally and spatially in the crystal and is proportional to the intensity of both beams, by changing the time at which each pulse arrives at the crystal the entire PL decay transient can be mapped out with a resolution roughly equal to the duration of the shorter gate pulse.
The general outline of a PL-UC setup is as follows: a single beam from a mode- locked oscillator or regenerative amplifier is split into two beams, one to pump the sample, and another to act as a gate pulse.
The pump beam is usually converted to a wavelength which coincides with the peak absorption of the sample. This is done with the aid of a harmonic generator or parametric oscillator/amplifier. The resulting higher-energy pump pulses are then focussed onto the sample, giving isotropic emission from a small spot. A fraction of this emission is collected and refocussed into a crystal with non- linear optical properties by a pair of off-axis parabolic mirrors. Reflective optics are usually chosen for PLUC spectroscopy in order to minimise any chirp of the PL pulse as this would lower the time resolution and shift the measured spectra.
Meanwhile, the gate beam is passed through an optical delay line and then focussed onto the same spot on the crystal as the PL.
When the two pulses meet at the crystal, sum-frequency generation occurs. Photons generated by this process are then separated from residual pump, PL, and gate photons, and measured by a sensitive detector.
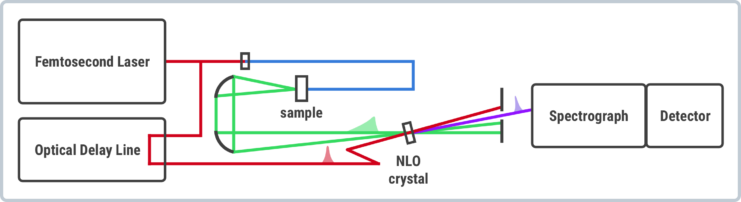
Disadvantages of the technique are the complexity of alignment, and the fact that critical phase-matching prevents an entire spectrum from being measured at once. In order to map out full spectra, the crystal must be tuned to measure different parts of the fluorescence spectrum sequentially.
Additionally, it is not possible to measure near the gate and pump wavelengths, as the sum-frequency photons are obscured by scattered harmonics of the intense gate pulse which are also generated in the crystal. This can be avoided however, if the gate pulse is tuned to a different wavelength by using an OPA.
Optical Kerr Gating Spectroscopy
Optical Kerr Gating (OKG) spectroscopy has been suggested as an alternative method to overcome some of the disadvantages of fluorescence upconversion spectroscopy. In particular, it allows measurement of the entire emission spectrum at once, while maintaining the high temporal resolution associated with optically gated measurement techniques.
The gating process in OKG makes use of the transient birefringence that is induced in many glass and liquid materials when exposed to a high enough electric field. This can be supplied in the form of a short, intense laser pulse when using a mode-locked laser and amplifier. If the fluorescence to be measured is sent through two crossed polarisers and a Kerr gating medium, then it will be blocked from reaching a detector when the gate pulse is not present. When it is present, however, the transient birefringence will temporarily distort the polarisation of the fluorescence from linear to elliptical, allowing some to pass through the second polariser and be detected. In this way, snapshots of the fluorescence spectrum can be measured with a time-resolution determined by the width of the gate pulse and the duration of the Kerr medium response.
Choosing a suitable material for the OKG experiment is one of the major challenges, as there is a trade-off between the efficiency of the optical Kerr effect and the response lifetime. In general, liquid Kerr media like CS2 and benzene have decent efficiencies, but since the Kerr effect in these materials is derived partially from molecular rotations, the response time and hence time resolution of the measurement is limited to 1.6 or 0.5 picoseconds respectively. Although the Kerr effect is less efficient in solid state crystals and glasses, materials such as YAG, SFL6 glass, or fused silica are commonly used, resulting in temporal resolutions between 85 and 250 ps.
The second major disadvantage is the high background associated with optical Kerr gating, which is not present in fluorescence upconversion. As there is no wavelength or spatial separation between the signal and the rest of the fluorescence pulse, leakage through the polarisers of even 10-5 can end up drowning out the signal. This is especially true when the sample’s fluorescence lifetime is longer than a nanosecond.
A third factor that must be considered is the response of the Kerr medium to the intense gate pulses that are required. In some cases, two-photon absorption of the gate pulse by the Kerr medium can result in fluorescence which downs out the signal, or optical damage to the medium. Care must also be taken to ensure that the gate pulses are not intense enough to cause other nonlinear effects in the Kerr medium such as supercontinuum generation, as these can also obscure the signal.
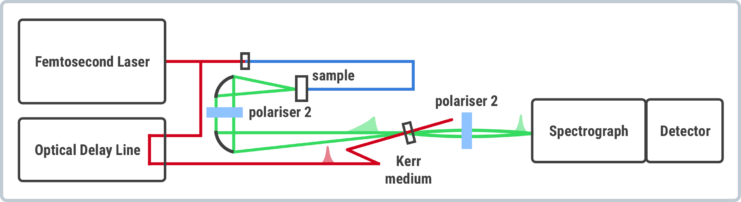
Streak Camera
Streak cameras are multicomponent systems which map spatial and temporal variations in input light into a 2D image which is then recorded by a sensitive CCD. This is achieved in 4 main steps – first, incident light passes through a horizontal slit and is imaged onto a photocathode. This results in the ejection of electrons by the photoelectric effect, with the number of electrons produced proportional to the light intensity. The second step is acceleration of electrons between a pair of electrodes, across which a field is swept from low to high field. This deflects electrons vertically with angles that depend on the field strength. As this deflection is time dependent, light pulses which arrived in the system later are deflected more strongly.
The third step is amplification of the spread-out electrons by 1000x in a micro- channel plate. Use of an MCP increases the sensitivity of the system while maintaining the spatial distribution from the previous step.
The final step is conversion of electrons back into photons with a phosphor screen and measurement of the 2D image with a CCD.
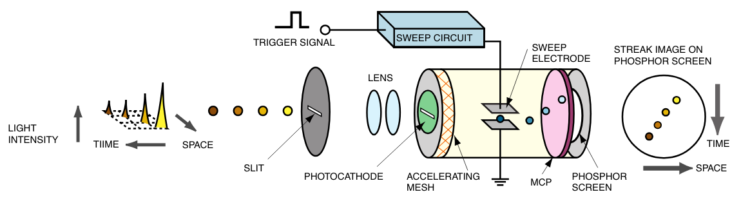
The main advantage of using streak cameras for spectroscopy is the very high time resolution that can be achieved with a single unit and a simple optical setup. 1 or 2 picoseconds is standard, and cutting-edge systems from Hamamatsu operating in the visible can even reach 100 femtoseconds which is comparable to upconversion. Additionally, since the field sweeps in the vertical direction and a monochromator can be used to spread the incident PL spectrum horizontally, the entire spatio-temporal fluorescence map can be measured in a single shot.
Unfortunately, such convenience does not come cheap!
Time Correlated Single Photon Counting (TCSPC)
TCSPC is a well-established method for measuring fluorescence lifetimes, with extremely high sensitivity and a time resolution of a few tens of picoseconds. The principle of operation is completely different to upconversion and the streak camera, as instead of directly measuring light intensity, it is the time difference between pulses from a detector and reference that is recorded. This offers two main benefits. Firstly, the time resolution of the experiment can be much smaller than the response of the detector being used, and secondly, the measured signal is free from any intensity noise in the excitation source.
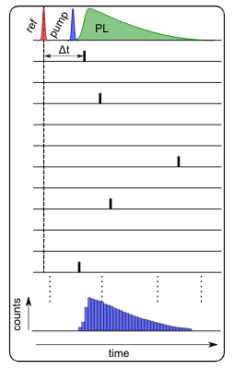
Each cycle, pulses from the laser are split in two. One is directed to a fast photodiode which generates a pulse that initiates the counting process. The other part of the pulse is used to excite the sample which then fluoresces. Emitted photons are collected and focussed onto a detector with fast response, usually a photomultiplier tube (PMT) or a single- photon avalanche diode (SPAD). The excitation intensity is kept low enough that a single photon results in an output pulse from the detector only once every few hundred cycles in order to avoid counting errors.
When a pulse from the detector reaches the counting electronics, the difference between arrival times of the reference and signal pulses is recorded and a single count added to a memory bin corresponding to this value. After repeating the process many thousands (or millions) of times, a histogram representing the transient PL intensity is developed.
TCSPC has the advantage of being both more sensitive than a streak camera. This comes at a cost of lower time resolution, and means that processes occurring on timescales of less than 50 ps are usually difficult to observe with this technique.
The maximum measurement window is longer than that of upconversion however, and is limited primarily by the repetition rate of the laser. For mode-locked Ti:Sapphire oscillators with repetition rates of 80 MHz, this corresponds to a time window of 12.5 nanoseconds. This can be easily extended by using a pulse picker, which blocks alternate pulses from the laser and allows longer fluorescence lifetimes to be measured.
Other pump sources with tuneable repetition rates are also available, including some supercontinuum lasers and pulsed diode lasers. These have pulse durations ranging from a few hundred femtoseconds to a few hundred picoseconds and are well suited to TCSPC. They have the added benefit of costing much less than a full modelocked system.
Another drawback of TCSPC is that the use of PMT and SPAD detectors means that it is essentially a single-wavelength measurement technique. In order to measure multiple wavelengths simultaneously, multiple detectors or a multi-anode PMT can be used. The number of channels on these detectors and hence the achievable spectral resolution is limited, however, when compared with the linear or 2D arrays that are commonly used with other techniques.
Intensified Charge Coupled Device
The intensified charge-coupled device is a device that works in a similar method to a streak camera, measuring the complete emission spectrum in a well-defined time window.
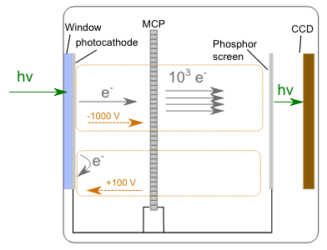
With both streak cameras and ICCDs, incident photons release electrons from a photocathode on entry into the system. Whereas in a streak camera the sweeping field deflects these electrons on their way to the MCP amplifier, in an ICCD an applied field between the photocathode and MCP either speeds electrons to the MCP or blocks their travel. This gating field acts as an electronic shutter, and the speed with which it can be turned on or off determines the time-resolution of the system. For many ICCDs this is limited to 2 to 5 nanoseconds, although some models are available with minimum shutter times of 200 picoseconds.
The opening and closing of the shutter can be delayed relative to a reference pulse either from the laser or from an external fast photodiode with a resolution of a few tens of picoseconds. By taking multiple shots with increasing delay times, the entire PL profile can be mapped out.
Copyright © 2019 Femtoresearch | All Rights Reserved